Fun with Latin Squares
Last year, our junior PRIMES STEP group studied Latin squares. We invented a lot of different types of Latin squares and wrote a paper about it, Fun with Latin Squares. Recall that a Latin square is an n by n table containing numbers 1 through n in every cell, so that every number occurs once in each row and column. In this post, I want to talk about anti-chiece Latin squares.
First, what’s a chiece? A chiece is a portmanteau word made out of two words, chess and piece, and, not surprisingly, it means a chess piece. Given a chiece, an anti-chiece Latin square is a Latin square such that any two cells, where our chiece can move from one cell to the other, according to the rules of chess, can’t contain the same number. Let’s see what this means.
Let’s start with rooks, which move along rows and columns. An anti-rook Latin square can’t have the same numbers repeating in any one row or column. Ha, anti-rook Latin squares are just Latin squares. Anti-bishop and anti-queen Latin squares can’t have the same numbers repeating on any diagonal.
Now, here is a picture of an anti-knight Latin square in which no two identical numbers are a knight’s move apart. This particular Latin square also forms a mini-Sudoku: not only does each row and column, but also each 2 by 2 corner region, contains all distinct numbers.
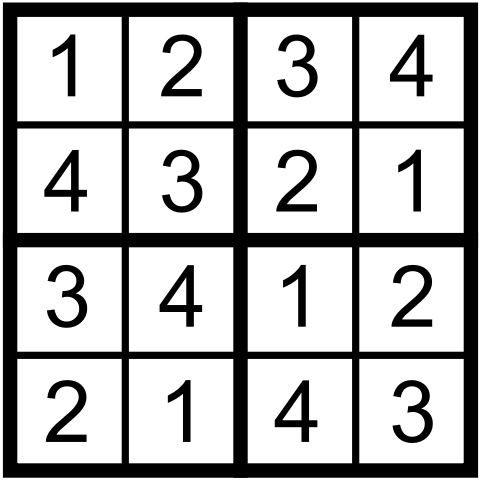
Consider all instances of some number, say 1, in an anti-chiece Latin square. If the board is n by n, we get n instances of non-attacking chieces. A famous math puzzle asks to place eight non-attacking queens on a standard chessboard. So the instances of any one particular number in an anti-queen Latin square solves the problem of placing n non-attacking queens on an n by n chessboard. Thus, building an anti-queen Latin square is more complicated than solving the non-attacking queens puzzle. The former requires filling the chessboard with n non-overlapping sets of non-attacking queens. The picture below gives an example of an anti-queen 5 by 5 Latin square.
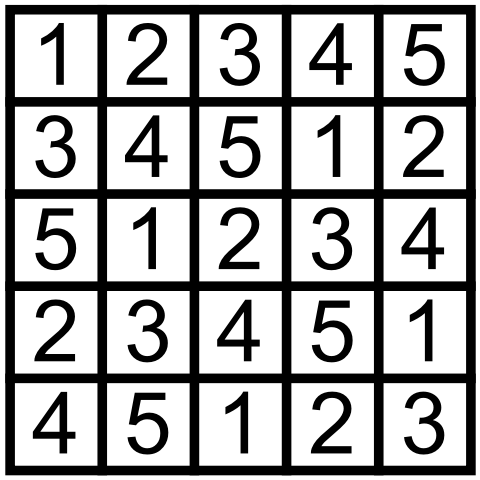
This square has some interesting properties. It can be formed by cycling the first row. It also happens to be one of the chiece Latin squares we study in our paper. A chiece Latin square is a Latin square such that for each number in a cell, there is another cell, a chiece’s move apart, containing the same number. You can check that our anti-queen Latin square is at the same time a knight Latin square.
I wonder, can anyone build an anti-queen Latin square on the standard 8 by 8 chessboard?
Share:

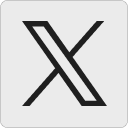



