The Art of Checking
I wrote a series of essays about AMC competitions:
- Metasolving AMC 8
- AMC, AIME, USAMO Contradiction
- Multiple Choice Proofs
- Should You Check Your Answers During Tests?
- To Guess or Not to Guess?
- Solving Problems with Choices
- How to Boost Your Guessing Accuracy During Tests
- Choices or No Choices
- Problem Design for Multiple Choice Questions
This essay is next in the series. Although it is not strictly about AMC, it should be useful during any test when you need to check your answers. There are several important rules which are helpful.
Rule 0. Checking is important. If wrong answers are punished, then correcting a mistake brings more points than solving a new problem. In addition, problems that were solved are often easier than problems yet to be solved, so finding a mistake might be faster than solving a new problem.
Rule 1. Your checking methods must be fast. The tests are generally timed. This means that in order to check your answers, you need to sacrifice your work on the next problem.
Rule 2. Customize how you check according to your strengths and weaknesses. For example, if you tend to jump to conclusions about what the question is going to be, and as a result answer your anticipated question instead of the one that is actually on the test, then when you are checking you should start reading the problem from the question. Or, if you usually make mistakes in geometry problems, you should allocate more time to geometry problems when you are checking. If you never make mistakes in arithmetic problems then you do not need to check those.
Rule 3. Mark problems that might need checking. If you do not have enough time to check all the problems, check only those you are not sure about.
Rule 4. Do not repeat your solution when you check. While solving the problem your brain often creates a pathway from start to finish. If on this pathway your brain decided to believe that two plus two is five, very often during checking, your brain will make the same mistake again. Because of that it is crucial to use other methods for checking than repeating your reasoning. In case you can’t find a way to check your answers using a different method and have to repeat your reasoning, you should repeat it in a different order.
This rule is so important, that I am providing some methods to change your brain pathway when you are checking your answers.
Plug in. Plugging in the answer you found is much faster than finding it. Use this method whenever possible. It is perfect for problems like this one below from 2004 AMC10-A:
What is the value of x if |x – 1| = |x – 2|?
Plug in an intermediate result. Sometimes you can’t plug in the answer, but you can plug in an intermediate result. In the following problem from 2004 AMC10-B you can plug in the number of nickels and dimes:
Patty has 20 coins consisting of nickels and dimes. If her nickels were dimes and her dimes were nickels, she would have 70 cents more. How much are her coins worth?
Calculate something else related to your answer. For example a negation. Here is a problem from 2004 AMC10-B:
How many two-digit positive integers have at least one 7 as a digit?
If you calculated the answer directly, to check it you may want to calculate the number of two-digit positive integers that do not contain 7.
Create an example. Sometimes you solve a problem by reasoning, but to check it you might create a particular example. Here is a problem from 2001 AMC10:
Let P(n) and S(n) denote the product and the sum, respectively, of the digits of the integer n. For example, P(23) = 6 and S(23) = 5. Suppose N is a two-digit number such that N = P(N) + S(N). What is the units digit of N?
If we denote the tens digit by a and the units digit by b, then N = 10a + b, P(N) = a*b, and S(N) = a + b. We get an equation a(b+1) = 10a, from which the answer is 9. To check the answer we do not need to repeat the reasoning. It is enough to check that 19 is the sum of the product of its digits plus the digits.
Here is another problem from 2001 AMC10:
Suppose that n is the product of three consecutive integers and that n is divisible by 7. Which of the following is not necessarily a divisor of n?
The list of choices is: 6, 14, 21, 28, 42. Your solution might go like this: the product of three consecutive numbers is divisible by 6. Hence, n is divisible by 42. So, the answer must be 28. To check you might consider a product of three consecutive numbers: 5*6*7=210 and see that it is not divisible by 4, hence it is not divisible by 28.
Rule 5. Embrace the partial check. It is very important to check your answers fast. Sometimes you can gain speed if you do not check the problem completely, but check it partially. For example, you can check that your answer is one of the two correct answers. There are many methods for partial checking.
Try an example. Sometimes an example doesn’t guarantee that your choice is correct, but it increases your confidence in your answer. Here is another problem from 2001 AMC10:
The sum of two numbers is S. Suppose 3 is added to each number and then each of the resulting numbers is doubled. What is the sum of the final two numbers?
The choices are: 2S + 3, 3S + 2, 3S + 6, 2S + 6, 2S + 12. You can reason that increasing each summand by 3, increases the sum by 6. After that doubling each summand increases the resulting sum twice, so the answer is 2S + 12. To check the answer you can use an example. Usually an example doesn’t guarantee the confirmation of your answer, but it might help you eliminate some of the wrong answers. For example, if you choose zero and zero as your initial two numbers, then S = 0, and your transformation brings the result to 12, which confirms your answer 2S + 12. In this particular case, a very easy specific example excluded all the wrong answers.
Divisibility. Sometimes it is faster to calculate the remainder of the answer by some number.
For example, look at the following problem from 2003 AMC10:
What is the units digit of 132003?
The choices are 1, 3, 7, 8, 9. We can immediately say that the answer must be an odd number.
Approximation check. One important example of a partial check is an approximation check. By estimating an approximate answer you might exclude most of the wrong answers. Consider this problem from 2001 AMC12:
How many positive integers not exceeding 2001 are multiples of 3 or 4 but not 5?
The divisibilities by 3, 4 or 5 shouldn’t correlate with each other. Approximately one third of those number are multiples of 3 and one quarter are multiples of 4. Let’s say that one twelfth are multiples of both 3 and 4. Hence, we estimate the portion of numbers that are multiples of 3 or 4 as 1/3 + 1/4 – 1/12 = 1/2. We have about 1,000 such numbers. The number of numbers that are, in addition, not divisible by 5, are less than that. So out of the given choice of (A) 768, (B) 801, (C) 934, (D) 1067, (E) 1167, we can immediately confirm that the answer is among the first three.
The methods above can be useful even if you do not have multiple choices. But if you do…
Rule 6. Use given choices as extra information. In the previous examples you saw how to use a partial check to exclude some of the choices. Here is a specific example from 2006 AMC10-A of how to exclude choices:
What non-zero real value for x satisfies (7x)14 = (14x)7?
The choices are: 1/7, 2/7, 1, 7, 14. If you solved the problem directly, to check it you can reason why other choices do not work. In this particular case it can be done very fast. 1/7 doesn’t work because the left part of the equation becomes 1 when the right is clearly not. 1 and 7 do not work because the left part is odd and the right is even; 14 doesn’t work because the left is clearly bigger than the right.
Rule 7. Use meta considerations. If you get into the mind of the designers you can better anticipate when you should check more thoroughly. Consider this problem from 2006 AMC10-A:
A digital watch displays hours and minutes with AM and PM. What is the largest possible sum of the digits in the display?
The most common mistake would be to assume that 12:59 supplies the largest sum, which is 17. But look at the choices: 17, 19, 21, 22, 23. When the designers are asking to find the largest number with some property, they assume that some students will make a mistake and chose a smaller number over a larger one. That means the designers would include this potential mistake among the choices. So the answer is extremely unlikely to be the smallest number on the list of choices. Thus, if you think the answer is 17, understanding how these problems are constructed should alert you to thoroughly check your answer. Indeed, the correct answer is 23 which corresponds to 9:59. Not surprisingly, it is the largest on the list of choices.
AMC 10/12 is coming on February 8 and HMMT on February 12. Happy checking.
Share:
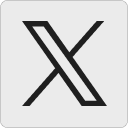



