Noisy Violinists
My PRIMES project last year, done with Rich Wang, was about violinists living in a hotel and being annoyed with each other. The project was suggested by Darij Grinberg and based on the following puzzle.
Puzzle. Consider a hotel with an infinite number of rooms arranged sequentially on the ground floor. The rooms are labeled from left to right with consecutive integers. A finite number of violinists are staying in the hotel with no more than one violinist in a room. Each night, two violinists staying in adjacent rooms get fed up with each other’s playing, and both request different rooms from the manager. The manager moves one of them to the nearest unoccupied room on the left and the other to the nearest unoccupied room on the right. This keeps happening every night as long as there are two violinists in adjacent rooms. Prove that this relocation will stop after a finite number of nights.
The project was not to solve the puzzle, and I won’t describe the solution, leaving it to my readers to ponder on their own. The project was to take this puzzle and see what we could discover. The relocation process in the puzzle is reminiscent of chip-firing, with a few differences.
Let me explain chip-firing in terms of violinists. The starting position would allow any number of violinists per room. Each night, two violinists in the same room will get annoyed with each other and request new rooms. There might be more violinists in that same room, but only two at a time are really annoyed. The manager will put one of them into the room on the left and the other into the room on the right, regardless of how crowded the rooms are.
In the chip-firing example, violinists always move to the next room. In our puzzle, they might need to go miles to a new room. And carry their luggage with them!
The original puzzle above implies that after several nights the relocations will stop, and violinists will enjoy the hotel in peace. This peaceful configuration is called a final state. Interestingly, depending on the order in which violinists are getting annoyed with each other, a starting configuration can result in different final states.
For example, consider the smallest interesting case, where only 3 violinists are staying in a hotel. We use 1 to describe a noisy room with a violinist and 0 to describe an empty room. Suppose the starting configuration is 0011100, where we ignore rooms far away from the noise. Depending on which two rooms have the complaining violinists on the first night, we can end up with a final state 0101001 or 1001010.
Initially, in the project, we looked at final states where we start with N violinists in consecutive rooms. We call such a configuration an N-clusteron. The example above describe the final states of a 3-clusteron. Here is an exercise for the reader: prove that in the final state, the gaps between occupied rooms have to be 1 or 2.
A final state can be described by the index of the leftmost occupied room and the sequence of gaps between occupied rooms. In the exercise above, it is easy to prove that the gaps must be size 1 or 2. In our paper, we proved a more refined statement: any final state has exactly one gap of size 2. Thus, a final state has two parameters: the index of the leftmost room and the location of the size 2 gap. For example, this means that in a final state, the span between the leftmost and the rightmost rooms, inclusive, is 2N.
As we continued exploring final states, we discovered something else. But first, I need to define the shadow of a final state. Let’s denote an occupied room with a one and an empty room with a zero. Then a final state corresponds to an infinite sequence of zeros and ones. But the interesting part of the final state is not infinite: it is a subsequence between the leftmost and rightmost ones, inclusive. We call this subsequence the shadow of the final state. In other words, the shadow describes the final state up to a translation and is completely defined by one parameter: the location of the 2-gap. In our example of a 3-clusteron, there are 2 final states with 2 distinct shadows. For larger clusterons, the situation is more interesting. There are 5 different possible final states for a 4-clusteron but only three distinct shadows. We discovered the following conjecture.
Conjecture. If we start from an N-clusteron and at each state uniformly select a move to perform from all possible moves, then the shadows of the final states are equiprobable.
You can find more details in our paper Ending States of a Special Variant of the Chip-Firing Algorithm. This conjecture is beautiful as it shows that there are hidden symmetries in this process of appeasing the fussy violinists. We didn’t prove this conjecture. Can you do it?
Share:
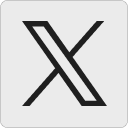



