How to Boost Your Guessing Accuracy During Tests
I promised to discuss how to improve the accuracy of your guessing at AMC 10/12, or other tests for that matter. There are two types of guessing. First, meta-guessing is when you do not look at the problem, but rather guess from just looking at the choices. Second, in the one I refer to as an educated-guess you do look at the problem. Instead of completely solving it, you try to deduce some information from the problem that will help you eliminate some of the choices. In this essay, I discuss both methods.
But first let me emphasize that solving problems is a more important skill than meta-guessing from a list of choices. Solving problems not only teaches you to think mathematically, but also increases your brain power. Spending time improving your meta-guessing skills can help you at multiple-choice tests and may give you insight into how test designers think, but this will not increase your math knowledge in the long run.
On the other hand, educated-guessing is a very useful skill to obtain. Not only can it improve your score during a test, the same methods can be applied to speed up the process of re-checking your answers before handing in the test. This skill will also come in handy when you start your research. Some problems in research are so difficult that even minor progress in estimating or describing your answer is beneficial.
Before discussing particular methods, let me remind you that AMC 10/12 is a multiple-choice competition with five choices for each question. The correct answer brings you 6 points. A wrong answer brings you 0 points, and not answering brings you 1.5 points. So if you randomly guess one of the five choices, your expected average score is 1.2 points, which is 0.3 less than your score for an unanswered question. Thus guessing is unprofitable on average.
However, if you can eliminate one choice, your expected average score becomes 1.5 points. In this case guessing doesn’t bring you points on average, but it does create some randomness in your results. For strategic reasons, you might prefer guessing, as I discussed in my earlier piece.
If you can eliminate two wrong choices, then guessing becomes profitable. A random guess out of three possibilities brings you 2 points, a better result than 1.5 points for an unanswered question. Even more, if you can eliminate three choices, then guessing will increase your score by 3 points on average.
Now that we’ve covered the benefits of excluding choices before guessing, I would like to discuss how to exclude choices by just looking at them. Let us take one of the problems from the 2002 AMC10B. Here are the choices: (-2,1), (-1,2), (1,-2), (2,-1), (4,4). The pair (4,4) is a clear outlier. I suggest that an outlier can’t be a correct choice. If (4,4) were the correct answer, then it would have been enough, instead of solving the problem, to use some intermediate arguments to choose it. For example, if you can argue that both numbers in the answer must be at least 2, or must be positive or be even, then you can get the correct answer without solving the problem. Any problem for which you can easily pick the correct answer without solving it is an unacceptably poor problem design. Thus, (4,4) can’t be the correct answer, and should be eliminated during guessing.
Let us look at a 2002 AMC10A problem with the following choices: 4/9, 2/3, 5/6, 3/2, 9/4. Test designers want to create choices that are plausible. They try to anticipate possible mistakes. In this set of choices, we can deduce that one of the mistakes that they anticipate is that students will confuse a number with its inverse. In this case 5/6 can’t be the correct answer. Otherwise, 6/5 would have been included as a choice. In another similar example from the 2000 AMC10 with choices -2, -1/2, 1/3, 1/2, 2, the designers probably hope that students will confuse numbers with their inverses and negations. Hence, we can exclude 1/3.
Sometimes the outlier might hint at the correct answer. Suppose you have the following list of choices: 2, 1/2π, π, 2π, 4π. The number 2 is an outlier here. Probably, the problem designers were contemplating that students might forget to multiply by π. In this case the likely correct answer would be 2π, because only from this answer can you get 2 by forgetting to multiply by π.
As an exercise, try to eliminate the wrong choices from the following set from a problem given at 2000 AMC10: 1/(2m+1), m, 1-m, 1/4m, 1/8m2.
AMC designers know all of these guessing tricks, so they attempt to confuse the competitors from time to time by going against common sense. For example, in a 2002 AMC10A problem the choices were: -5, -10/3, -7/3, 5/3, 5. I would argue that -7/3 is a clear outlier because all the others are divisible by 5. Furthermore, there is no point in including so many answers with 3 in the denominator unless there is a 3 in the denominator of the correct answer. So I would suggest that one of -10/3 and 5/3 must be the answer. My choice would be 5/3, as there is a choice of 5 which I would assume is there for students who forgot to divide by 3. As I said the designers are smart and the actual answer is -10/3. They would have tricked me on this problem.
One of the best ways to design a multiple choice question is to have an arithmetic progression as a list of choices. There is no good way to eliminate some choices from 112, 113, 114, 115, 116. Unfortunately for people who want to get an advantage by guessing, many of the problems at AMC have an arithmetic progression as their choices.
Now that we’ve seen the methods of meta-guessing, let’s look at how to make an educated guess. Let us look at problem 1 in 2003 AMC10A.
What is the difference between the sum of the first 2003 even counting numbers and the sum of the first 2003 odd counting numbers?
Without calculations we know that the answer must be odd. Thus, we can immediately exclude three out of five choices from the given choices of 0, 1, 2, 2003, 4006. Parity consideration is a powerful tool in eliminating wrong answers. Almost always you can decide the parity of the answer much faster than you can calculate the answer. Similar to using parity, you can use divisibility by other numbers to have a fast elimination. Here is a problem from 2000 AMC10:
Mrs. Walter gave an exam in a mathematics class of five students. She entered the scores in random order into a spreadsheet, which recalculated the class average after each score was entered. Mrs. Walter noticed that after each score was entered, the average was always an integer. The scores (listed in ascending order) were 71, 76, 80, 82, and 91. What was the last score Mrs. Walter entered?
The first four numbers entered must be divisible by 4. The total of the given numbers is divisible by 4. Hence, the last number must also be divisible by 4. This reasoning eliminates three out of five choices.
Another powerful method is a rough estimate. Let us look at the next problem in 2003 AMC10A.
Members of the Rockham Soccer League buy socks and T-shirts. Socks cost $4 per pair and each T-shirt costs $5 more than a pair of socks. Each member needs one pair of socks and a shirt for home games and another pair of socks and a shirt for away games. If the total cost is $2366, how many members are in the League?
If we notice that the cost per person is more than $25, we can conclude that there are less than a hundred members in the League. Given the choices of 77, 91, 143, 182, and 286, we immediately can eliminate three of them.
Another method is to use any partial knowledge that you may have. Consider this problem from 2003 AMC10A:
Pat is to select six cookies from a tray containing only chocolate chip, oatmeal, and peanut butter cookies. There are at least six of each of these three kinds of cookies on the tray. How many different assortments of six cookies can be selected?
You might remember that there is a formula for this. Even if you do not remember the exact formula, you might still have a vague memory that the answer must be a binomial coefficient that somehow uses the number of cookies and the number of flavors. Looking at the choices — 22, 25, 27, 28, 29 — you can see that the only choice that appears in the first 10 rows of the Pascal’s triangle is 28. So you should go with 28.
It is easy to talk about easy problems; let us see what we can do about difficult ones. Consider the last problem on 2003 AMC10A:
Let n be a 5-digit number, and let q and r be the quotient and the remainder, respectively, when n is divided by 100. For how many values of n is q+r divisible by 11?
The choices are 8180, 8181, 8182, 9000, 9090. They can be naturally split into two groups: three choices below 9000 and the rest. By my rules of removing outliers the group of numbers below 9000 seems the more promising group. But I would like to discuss how to approximate the answer. There is no reason to believe that there is much correlation between remainders by 100 and divisibility by 11. There is a total of 90,000 5-digit numbers; among those numbers, approximately 90,000/11 = 8182 is divisible by 11, so we should go with the group of answers close to 8182.
Another way of thinking about this problem is the following. There are 900 different quotients by 100 to which we add numbers between 0 and 99. Thus for every quotient our sums are a set of 100 consecutive numbers. Out of 100 consecutive numbers usually 9, and rarely 10, are divisible by 11. Hence, the answer has to be less than 9000.
Sometimes methods you use for guessing can bring you the answer. Here is a problem from 2001 AMC12:
What is the product of all odd positive integers less than 10000? (A) 10000!/5000!2, (B) 10000!/25000, (C) 9999!/25000, (D) 10000!/(250005000!), (E) 5000!/25000.
For a rough estimate, I would take a prime number and see in what power it belongs to the answer. It’s simplest to consider a prime number p that is slightly below 5000. Then p should appear as a factor in the product of all odd positive integers below 10000 exactly once. Now let us look at the choices. Number p appears in 5000! once and in 10000! twice (as p and 2p). Hence, it appears in (A) zero times, and twice each in (B) and (C). We also can rule out (E) as the product of odd numbers below 10000 must be divisible by primes between 5000 and 10000, but 5000! doesn’t contain such primes. Thus the answer must be (D).
The method I just described won’t produce the formula. But the ideas in this method allow you to eliminate all the choices except the right one. Moreover, this method provides you with a sanity check after you derive the formula. It also helps to build your mathematical intuition.
I hope that you will find my essays about AMC useful. And good luck on February 9!
Share:
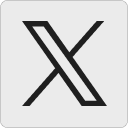



