Geometry Puzzles from the 35-th Lomonosov Tournament
Problem 1. It is true that it is possible to put positive numbers at the vertices of a triangle so that the sum of two numbers at the ends of each side is equal to the length of the side?
This looks like a simple linear algebra question with three variables and three equations. But it has a pretty geometrical solution. What is it?
Problem 2. Prove that it is possible to assign a number to every edge of a tetrahedron so that the sum of the three numbers on the edges of every face is equal to the area of the face.
Again we have six sides and four faces. There should be many solutions. Can you find a geometric one?
Share:
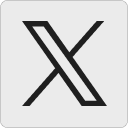




Your Deal is Better than my Deal
Do you know how to cut a cake? I mean, mathematically. There is a whole area of mathematics that studies cake cutting.
Mathematicians usually assume that each person has their own idea of what is the best part of the cake. Suppose three sisters are celebrating the New Year by having a cake. Anna likes only icing, Bella likes only chocolate chips, and Carol likes only pieces of walnuts mixed into the cake. Mathematicians want to cut cakes fairly. But what is fair? Fair is fair, right? Wrong. There are several different notions of fair cake division.
There is a proportionate division. In such a division every sister gets at least one-third of her value of the cake. This seems fair. Let’s see an example. Anna gets one third of the icing, Bella gets one third of the chocolate chips, and Carol gets everything else. This is a fair proportionate division. Each of the sisters believes that she got at least one-third of the cake, in their own value. But it doesn’t seem quite fair.
There is a stronger notion of fairness. It is called envy-free. In this division each sister gets at least one-third of the cake and, in addition, none of the three sisters would improve their value by swapping pieces. That means, if Anna wants only icing, not only does she get at least one-third of the icing, but also no one else gets more icing than Anna. The previous example of the proportionate division is not envy-free. Carol got two-thirds of the icing, so Anna would want to switch with her.
Let’s try a different division. Anna gets one third of the icing, Bella gets the chocolate chips and another third of the icing, and Carol gets all the walnuts and another third of the icing. Formally, this is envy-free cake cutting. But poor Anna. What do you think Anna feels when she sees the smiles of contentment on the faces of her sisters? Whoever invented the name doesn’t understand envy. Anna got one-third of the cake by her value, but the other sisters got the whole cake!
Luckily mathematicians understand this conundrum. So they invented another name for a cake division. They call a division equitable if everyone values all the pieces the same. So the division above is envy-free but not equitable. Let’s try again. Let’s give each sister one-third of all the components of the cake. This division is very good mathematically: it is proportionate, envy free, and equitable. By the way, envy-free division is always proportionate. This division seems fair. But is it a good division?
There is another term here: Pareto-efficient division means that it is impossible to make one person feel better, without making another person feel worse. All divisions above are not Pareto-efficient. Moving some icing from Carol to Anna, doesn’t decrease the value for Carol, but increases the value for Anna.
There is an even better way to divide the cake. We can give the icing to Anna, the walnuts to Bella, and the chocolate chips to Carol. This division is envy-free, equitable, and Pareto-efficient. It is perfect. Mathematicians even have a word for it. They call it a perfect division.
Mathematically this division is perfect. Unfortunately, sisters are not. I know an Anna who would still envy Bella.
Share:
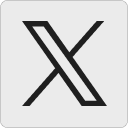




The Reuleaux Tetrahedron
Why are manhole covers round? The manhole covers are round because the manholes are round. Duh! But the cute mathematical answer is that the round shapes are better than many other shapes because a round cover can’t fall into a round hole. If we assume that the hole is the same shape as the cover but slightly smaller, then it is true that circular covers can’t fall into their holes. But there are many other shapes with this property. They are called the shapes of constant width.
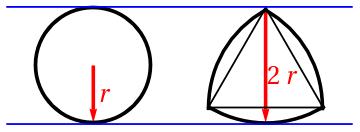
Given the width, the shape with the largest area is not surprisingly a circle. The shape with the smallest area and a given constant width is a Reuleaux Triangle. Here is how to draw a Reuleaux triangle. Draw three points that are equidistant from each other at distance d. Then draw three circles of radius d with the centers at given points. The Reuleaux triangle is the intersection of these three circles.
Can we generalize this to 3d? What would be an analogue of a Reuleaux Triangle in 3d? Of course, it is a Reuleaux Tetrahedron: Take four points at the vertices of a regular tetrahedron; take a sphere at each vertex with the radius equal to the edge of the tetrahedron; intersect the four spheres.
Is this a shape of the constant width? Many people mistakenly think that this is the case. Indeed, if you squeeze the Reuleaux tetrahedron between two planes, one of which touches a vertex and another touches the opposite face of the curvy tetrahedron, then the distance between them is equal to d: the radius of the circle. This might give you the impression that this distance is always d. Not so. If you squeeze the Reuleaux tetrahedron between two planes that touch the opposite curvy edges, the distance between these planes will be slightly more than d. To create a shape of constant width you need to shave off the edges a bit.
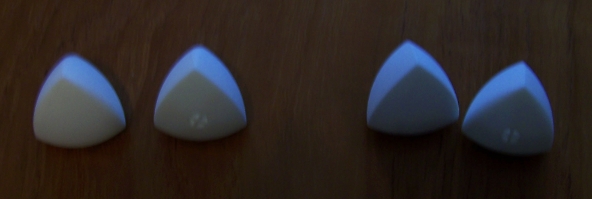
Theoretically you can shave the same amount off every edge to get to a surface of constant width. But this is not the cool way to do it. The cool way is to shave a bit more but only from one edge of the pair of opposite edges. You can get two different figures this way: one that has three shaved edges forming a triangle, and the other, where three shaved edges share a vertex. These two bodies are called Meissner bodies and they are conjectured to be shapes of the constant width with the smallest volume.
On the picture I have two copies of a pair of Meissner bodies. The two left ones have three edges that share a vertex shaved off. The very left shape gives a top view of this vertex and the solid next to it has its bottom with holes looking forward. The two shapes on the right show the second Meissner body in two different positions.
I recently discovered a TED-Ed video about manhole covers. It falsely claims that the Reuleaux tetrahedron has constant width. I wrote to TED-Ed, to the author, and posted a comment on the discussion page. There was no reaction. They either should remove the video or have an errata page for it. Knowingly keeping a video with an error that is being viewed by thousands of people is irresponsible.
Share:
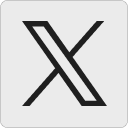




Who is Guilty?
I am running a PRIMES-STEP program for middle school students, where we try to do research in mathematics. In the fall of 2015 we decided to study the following topic in logic.
Suppose there is an island where the following four types of people live:
- Absolute truth-tellers always tell the truth.
- Partial truth-tellers tell the truth with one exception: if they are guilty, they will say that they are innocent.
- Absolute liars always lie.
- Responsible liars lie with one exception: if they are guilty, they will say that they are guilty.
See if you can solve this simple logic puzzle about people on this island.
It is known that exactly one person stole an expensive painting from an apartment. It is also known that only Alice or Bob could have done it. Here are their statements:
Alice: I am guilty. Bob is a truth-teller.
Bob: I am guilty. Alice stole it. Alice is the same type as me.
Who stole the painting and what types are Alice and Bob?
My students and I discovered a lot of interesting things about these four types of people and wrote a paper: Who Is Guilty?. This paper contains 11 cute logic puzzles designed by each of my 11 students.
I envied my students and decided to create two puzzles of my own. You have already solved the one above, so here is another, more difficult, puzzle:
Share:A bank was robbed and a witness said that there was exactly one person who committed the robbery. Three suspects were apprehended. No one else could have participated in the robbery.
Alice: I am innocent. Bob committed the crime. Bob is a truth-teller.
Bob: I am innocent. Alice is guilty. Carol is a different type than me.
Carol: I am innocent. Alice is guilty.
Who robbed the bank and what types are the suspects?

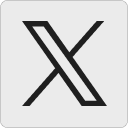




A Travel Puzzle
The following cute puzzle of unknown origins was sent to me by Martina Balagovic and Vincent van der Noort:
Share:A car travels from A to B and back again. When going uphill it goes 56 km an hour, when going downhill it goes 72 km an hour and while driving on flat surface it goes 63 km an hour. Getting from A to B takes 4 hours and getting back (over the same road) takes 4 hours and 40 minutes.
What is the distance between A and B?

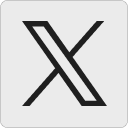




Really Big Numbers
I received a book Really Big Numbers by Richard Schwartz for review. I was supposed to write the review a long time ago, but I’ve been procrastinating. Usually, if I like a book, I write a review very fast. If I hate a book, I do not write a review at all. With this book I developed a love-hate relationship.
Let me start with love. I enjoyed reading the first 80 pages. The pictures are great, and some explanations are very well thought out. Plus, I haven’t thought much about really big numbers, so the book helped me understand them. I was impressed with how this book treats very difficult ideas with simple explanations and illuminating images. I was captivated by it.
Now to hate. I had two problems with this book: one pedagogical and the other mathematical.
The pedagogical issue. The beginning of the book is suitable for small children. Most of the book is suitable for advanced middle-schoolers who like mathematics. The last part is very advanced. Is it a good idea to show children a book that looks like a children’s book, but which soon becomes totally out of their reach? Richard Schwartz understands it and says many times that pieces of this book might be read several years apart. Several years? What child is ready to wait several years to finish a book? How would children feel about the book and about numbers when no matter how hard they try, they cannot understand the end of the book?
As a reviewer, I can’t recommend the full book for kids who are not ready to grasp the notion of the Ackermann function or arrow notation. Even if the child is capable of understanding these ideas, there are mathematical issues that would prevent me from recommending it.
The mathematical issue. Let me start by explaining the notion of plex. We call an n-plex a number that is equal to 10n. For example, 2-plex means 102 which is 100, and 10-plex means 1010. The fun part starts when we plex plexes. The number n-plexplex means 10 to the power n-plex which is 10(1010). We can continue plexing: n-plexplexplex means 10 to the power n-plexplex. When you are hunting for really big numbers, it is easier to write the number of plexes rather than writing plexes after plexes. Richard Schwartz introduces the following notation to help visualize the whole thing. He puts numbers in a square. Number n in a square means 1-plexed n times. For example, 2 inside a square means 1010. Ten inside a square is 1-plexplexplexplexplexplexplexplexplexplex.
We can start nesting squares. For example, 2 inside a square means 1-plex-plex or 1010. Let’s add a square around it: 2 inside two squares means 1010 inside a square, which equals 1 plexed 1010 times. To denote 10 nested in n squares Richard Schwartz uses the next symbol: n inside a pentagon. For example, 1 inside a pentagon is 10 inside a square. I wrote this number in the previous paragraph: it is 1-plexplexplexplexplexplexplexplexplexplex. Similarly, n inside a hexagon means 10 inside n nested pentagons. We can continue this forever: n inside a k-gon is 10 inside k nested (k-1)-gons.
What bothers me is why a square? Why not a triangle? If we adopt this scheme, what is the meaning of a number in a triangle? Let’s try to unravel this. Following Richard Schwartz’s notation we get that n inside a square is the same as 10 inside n nested triangles. What do we do n times with 10 to get to 1 plexed n times? 1-plexed n times is 10 plexed n-1 times. There is a disconnect in notation here. For example, 10 in two nested triangles should mean 2 inside a square that is 1010. 10 inside one triangle should mean 1 inside a square which is 10. This doesn’t make any sense.
I started googling around and discovered the Steinhaus–Moser notation. In this notation a number n in a triangle means nn. A number n in a square means the number n inside n nested triangles. A number n in a pentagon means the number n inside n nested squares. And so on. This makes total sense to me. If we move down the number of sides, we can say that the number n inside a 2-gon means n times n and the number n inside a 1-gon means n. This is perfect.
Schwartz changed the existing notation in two places. First he made everything about 10. This might not be such a bad idea except his 10 inside a square doesn’t equal 10 inside a square in Steinhaus–Moser notation. In Steinhaus–Moser notation 10 inside a square means 10 plexed 10 times. The author removed one of the plexes. He made 10 inside the square to mean 1 plexed 10 times, and as a result it stopped working.
Even though the first 83 pages are delightful and the pictures are terrific, the notation doesn’t work. What a pity.
Share:
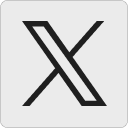




A River-Crossing Puzzle
I have solved too many puzzles in my life. When I see a new one, my solution is always the intended one. But my students invent other ideas from time to time and teach me to think creatively. For example, I gave them this puzzle:
Two boys wish to cross a river, but there is a single boat that can take only one boy at a time. The boat cannot return on its own; there are no ropes or similar tricks; yet both boys manage to cross the river. How?
Here is what my inventive students came up with:
- There was another person on the other side of the river who brought the boat back.
- There was a bridge.
- The boys can swim.
- They just wanted to cross the river and come back, so they did it in turns.
And here is my standard solution: They started on different sides of the river.
I gave a talk about thinking inside and outside the box at the Gathering for Gardner conference. I mentioned this puzzle and the inventiveness of my students. After the conference a guy approached me with another answer which is now my favorite:
- They wait until the river freezes over and walk to the other side.

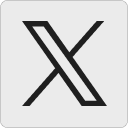




An Orthogonal Quadrangle
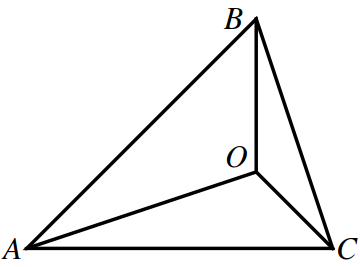
Consider a triangle with vertices A, B, and C. Let O be its orthocenter. Let’s connect O to the vertices. We get six lines: three sides of the triangle and three altitudes. These six lines are pair-wise orthogonal: AO ⊥ BC, BO ⊥ AC, and CO ⊥ AB.
It is easy to see that A is the orthocenter of the triangle OBC, and so on: each vertex is the orthocenter of the triangle formed by the other three. We say that these four points form an orthocentric system.
I heard a talk about this structure at the MOVES 2015 conference by Richard Guy. What I loved in his talk was his call to equality and against discrimination. The point O plays the same role as the other three points. It should be counted. Richard Guy suggested calling this system an orthogonal quadrangle. I am all for equality. This is not a triangle, this is a quadrangle!
Share:
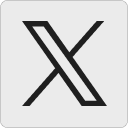




The Battle I am Losing
I want the world to be a better place. My contribution is teaching people to think. People who think make better decisions, whether they want to buy a house or vote for a president.
When I started my blog, I posted a lot of puzzles. I was passionate about not posting solutions. I do want people to think, not just consume puzzles. Regrettably, I feel a great push to post solutions. My readers ask about the answers, because they are accustomed to the other websites providing them.
I remember I once bought a metal brainteaser that needed untangling. The solution wasn’t included. Instead, there was a postcard that I needed to sign and send to get a solution. The text that needed my signature was, “I am an idiot. I can’t solve this puzzle.” I struggled with the puzzle for a while, but there was no way I would have signed such a postcard, so I solved it. In a long run I am glad that the brainteaser didn’t provide a solution.
That was a long time ago. Now I can just go on YouTube, where people post solutions to all possible puzzles.
I am not the only one who tries to encourage people to solve puzzles for themselves. Many Internet puzzle pages do not have solutions on the same page as the puzzle. They have a link to a solution. Although it is easy to access the solution, this separation between the puzzle and its solution is an encouragement to think first.
But the times are changing. My biggest newest disappointment is TED-Ed. They have videos with all my favorite puzzles, where you do not need to click to get to the solution. You need to click to STOP the solution from being fed to you. Their video Can you solve the prisoner hat riddle? uses my favorite hat puzzle. (To my knowledge, this puzzle first appeared at the 23-rd All-Russian Mathematical Olympiad in 1997.) Here is the standard version that I like:
A sultan decides to give 100 of his sages a test. He has the sages stand in line, one behind the other, so that the last person in line can see everyone else. The sultan puts either a black or a white hat on each sage. The sages can only see the colors of the hats on all the people in front of them. Then, in any order they want, each sage guesses the color of the hat on his own head. Each hears all previously made guesses, but other than that, the sages cannot speak. Each person who guesses the color wrong will have his head chopped off. The ones who guess correctly go free. The rules of the test are given to them one day before the test, at which point they have a chance to agree on a strategy that will minimize the number of people who die during this test. What should that strategy be?
The video is beautifully done, but sadly the puzzle is dumbed down in two ways. First, they explicitly say that it is possible for all but one person to guess the color and second, that people should start talking from the back of the line. I remember in the past I would give this puzzle to my students and they would initially estimate that half of the people would die. Their eyes would light up when they realized that it’s possible to save way more than half the people. They have another aha! moment when they discover that the sages should start talking from the back to the front. This way each person sees or hears everyone else before announcing their own color. Finally, my students would think about parity, and voilà, they would solve the puzzle.
In the simplified adapted video, there are no longer any discoveries. There is no joy. People consume the solution, without realizing why this puzzle is beautiful and counterintuitive.
Nowadays, I come to class and give a puzzle, but everyone has already heard the puzzle with its solution. How can I train my students to think?
Share:
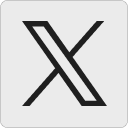



